Smart electricity meters give building managers insight into and control over energy use. Littelfuse makes the solid state relays,

SIDACtor®保护晶闸管
SIDACtor保护晶闸管可防止雷击和交流电源交叉造成的瞬态电压浪涌造成的损坏。浪涌保护器件还可保护人员和电子设备免受人机交互产生的静电放电的影响。这些用于交流电的专用硅二极管(SIDAC)与LED保护器一起成为Littelfuse提供的电路保护产品。查阅我们的产品目录 为您的宽带、基带、蜂窝、DS1、LCAS、SLIC、语音或照明应用找到解决方案。
下属的子类别 SIDACtor®保护晶闸管
SIDACtor® 保护晶闸管零件
SIDACtor®保护晶闸管 应用
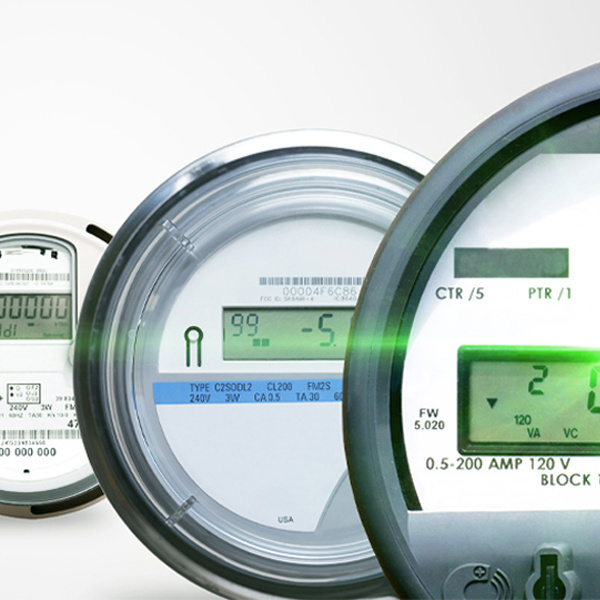
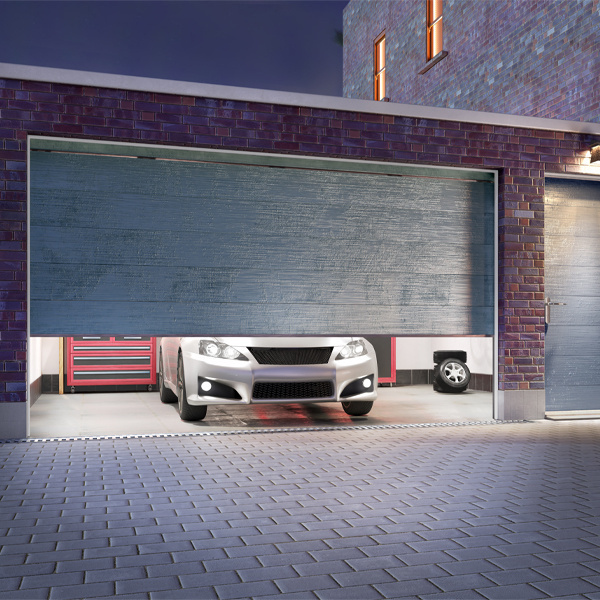
Automatic doors and gates make buildings more accessible and improve security. Consult this block diagram to find Littelfuse components that help
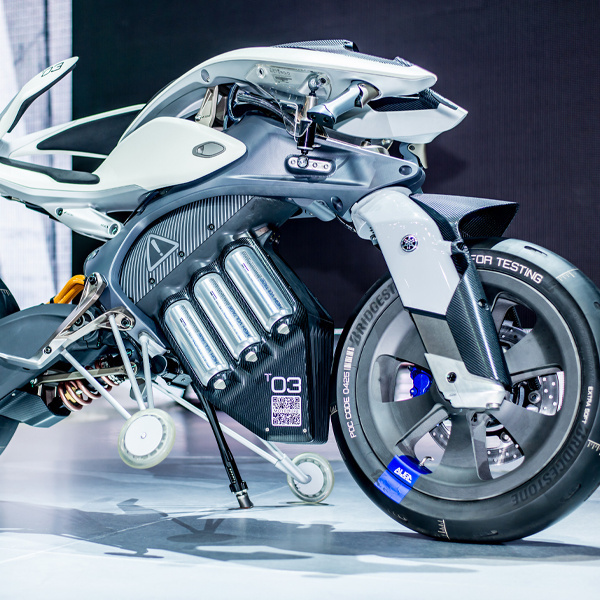
Littelfuse为两轮和三轮电动车的储能设备提供过流和过压保护。 This block diagram
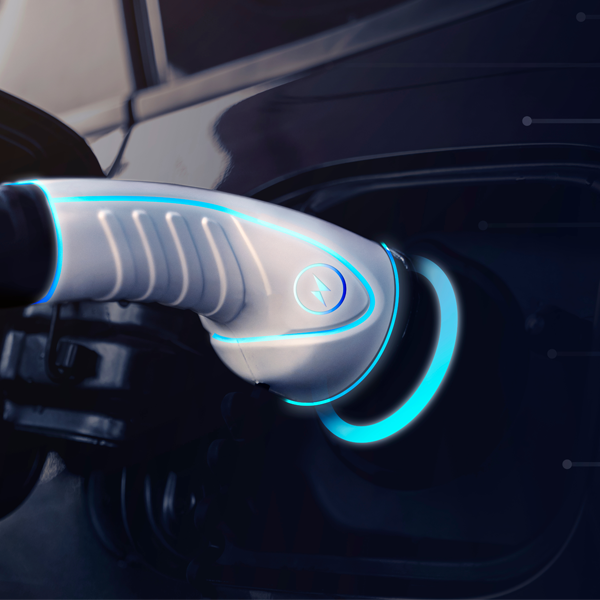
Depend on Littelfuse to deliver reliable electronics solutions for each component of a wireless charging system for electric cars, buses, and
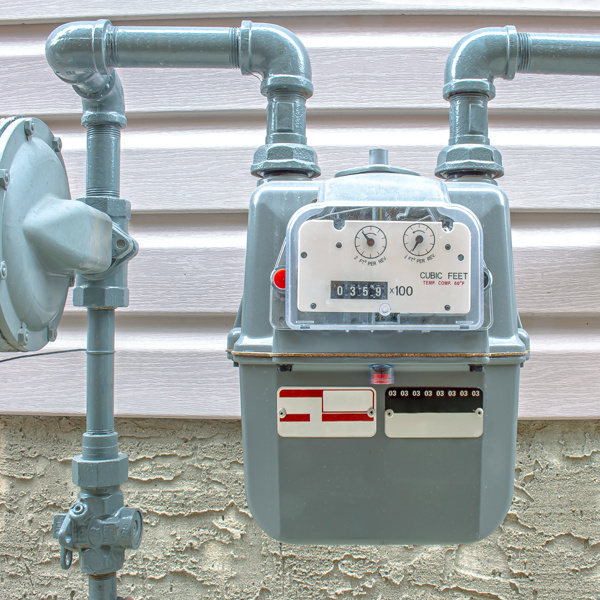
Smart gas and water meters promise to give building managers important tools to lower utility bills and increase safety. The flow meters can only
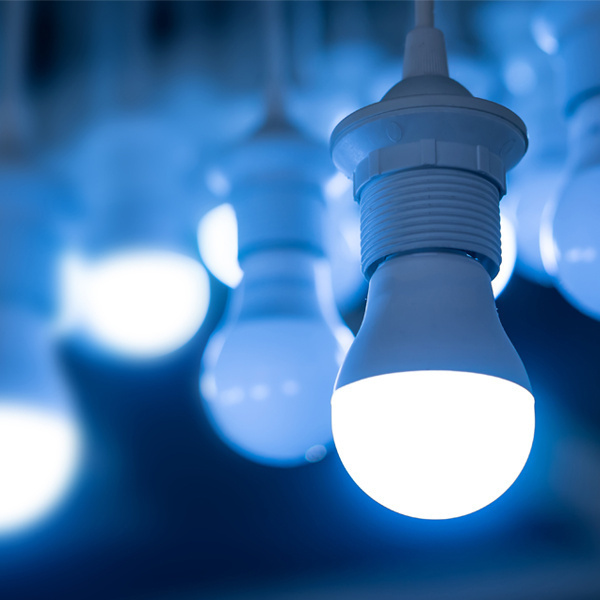
Industrial, outdoor, and residential LED lighting systems need different arrangements of electronic components. Block diagrams show how Littelfuse
SIDACtor® 保护晶闸管资源
SIDACtor® 保护晶闸管信息中心
宽带优化保护
宽带优化系列产品专注于满足宽带设备的性能和监管要求。宽带优化系列及其广泛的解决方案为应用提供了满足DSL设备(最高VDSL)以及以太网(最高1000baseT)独特保护需求的选项。优化过程是通过使用专属和专利的方法来实现的,可以最大程度地降低设备电容对宽带信号的负面影响。宽带优化系列提供过压保护解决方案,有助于应用设备遵守Telcordia GR-1089 问题4以及ITU-T建议K.20、K.21、K.44和K.45。
SLIC保护
SLIC系列产品专注于满足SLIC(用户线路接口电路)芯片组的独特保护需求。该系列提供固定电压和Battrix®电池跟踪保护解决方案,能够保护SLIC器件免受雷击和交流电流交叉引起的瞬态影响。SLIC系列提供了一种过压保护解决方案,有助于应用设备遵守Telcordia GR-1089 问题4以及ITU-T建议K.20、K.21、K.44和K.45。
LCAS保护
LCAS系列产品主要满足线路接入交换机(LCAS)的特殊保护需求。此系列采用的是LCAS器件专用的特有非对称设计。LCAS系列提供了一种过压保护解决方案,有助于应用设备遵守Telcordia GR-1089 问题4以及ITU-T建议K.20、K.21、K.44和K.45。
基带保护
基带系列产品专注于满足语音、调制解调器和DS1等基带电信设备的性能和监管要求。此类产品可提供过压保护解决方案,有助于使应用设备符合Telcordia GR-1089 Issue 4、ITU-T建议K.20、K.21、K.44、K.45和TIA-968-A。
高浪涌电流保护
高浪涌电流产品是一个独特的非常强大的固态保护器件系列,适用于高暴露环境。此系列中包括专为提供基础保护而设计的产品,如电池和TO-220器件。高浪涌电流系列还拥有能够在极端条件下达到5 kA 8/20μs的器件。为了满足增强的次级保护要求,我们可提供能够达到1000A 2/10μs的D级器件,采用DO-214封装。高浪涌电流保护系列提供了一种过压保护解决方案,有助于应用设备遵守Telcordia GR-1089 问题4以及ITU-T建议K.20、K.21、K.44和K.45。
SIDACtor系列应用选择表
电信应用 | Broadband Optimized™保护 | SLIC保护 | 基带保护(语音-DS1) | LCAS保护 | 高浪涌电流保护 |
---|---|---|---|---|---|
ADSL | - | - | |||
ADSL2/2+ | - | ||||
VDSL | - | ||||
VDSL2 | - | ||||
HDSL2/4 | - | - | |||
ISDN | - | - | |||
以太网10/100/1000BaseT | - | ||||
PoE | - | ||||
VoIP FXO | - | ||||
VoIP FXS | - | ||||
反向振铃SLIC | - | ||||
正向及反向振铃SLIC | - | ||||
LCAS继电器 | - | ||||
POTS电话——有绳及无绳 | - | ||||
MDC调制解调器 | - | ||||
PCI调制解调器 | - | ||||
多功能打印机—传真机 | - | ||||
T1/E1/J1 (DS1) | - | - | |||
安全系统 | - | ||||
初级保护模块 | - | ||||
次级保护模块——条状保护器 | - | - | |||
小对数电缆安装 | - | ||||
CATV功率放大器 | - | ||||
基站 | - |
选择SIDACtor器件时,请使用以下条件。
断态电压(VDRM)
SIDACtor器件的VDRM必须大于SIDACtor器件所保护电路的最大工作电压。
Example 1: For a POTS (plain old telephone service) application, convert the maximum operating ring voltage (150 VRMS) to a peak voltage, and add the maximum DC bias of the central office battery:
- 150 VRMS v2 + 56.6 VPK = 268.8 VPK
- ∴ VDRM > 268.8 V
Example 2: For an ISDN application, add the maximum voltage of the DC power supply to the maximum voltage of the transmission signal (for U.S. applications, the U-interface will not have a DC voltage, but European and Japanese ISDN applications may):
- 150 VPK + 3 VPK = 153 VPK
- ∴ VDRM > 153 V
开关电压(VS)
SIDACtor器件的VS值应等于或小于所保护元件的额定瞬时峰值电压。
Example 1: VS = VRelay Breakdown
Example 2: VS = SLIC VPK
峰值脉冲电流(IPP)
For circuits that do not require additional series resistance, the surge current rating (IPP) of the SIDACtor device should be greater than or equal to the surge currents associated with the lightning immunity tests of the applicable regulatory requirement (IPK):
- IPP = IPK
For circuits that use additional series resistance, the surge current rating (IPP) of the SIDACtor device should be greater than or equal to the available surge currents associated with the lightning immunity tests of the applicable regulatory requirement (IPK(available)):
- IPP = IPK(可用)
The maximum available surge current is calculated by dividing the peak surge voltage (VPK) by the total circuit resistance (RTOTAL):
- IPK(可用)= VPK/RTOTAL
For longitudinal surges (TIP-Ground, Ring-Ground), RTOTAL is calculated for both TIP and ring:
- RSOURCE = VPK/IPK
- RTOTAL = RTIP + RSOURCE
- RTOTAL = RRING + RSOURCE
For metallic surges (TIP-Ring):
- RSOURCE = VPK/IPK
- RTOTAL = RTIP + RRING + RSOURCE
Example 1: A modem manufacturer must pass the Type A surge requirement of TIA-968-A without any series resistance.
- IPK = 100 A,10x560 μs
- IPP = 100 A,10x560 µs
- 因此,应选择“B”级或“C”级SIDACtor器件。
Example 2: A line card manufacturer must pass the surge requirements of GR 1089 with 30 O on TIP and 30 O on ring.
- IPK = 100 A,10x1000 μs
- VPK = 1000 V
- RSOURCE = VPK/IPK = 10 O
- RTOTAL = RSOURCE + RTIP = 40 O
- IPK(可用)= VPK/RTOTAL = 1000 V/40 O
- ∴ IPP = 25 A
保持电流(IH)
由于TIA-968-A 4.4.1.7.3规定,注册的终端设备在短路的情况下,每个导体的直流电流不得超过140 mA,因此SIDACtor器件的保持电流被设定为150 mA。
就具体的设计标准而言,SIDACtor器件的保持电流(IH)必须大于工作和短路条件下可提供的直流电流。
断态电容(CO)
假定插入损失的临界点是原信号值的70%,SIDACtor器件可用于传输速度高达30 MHz的大多数应用中。对于大于 30 MHz的传输速度,强烈推荐使用新的MC系列。
由于服务中断和网络设备故障会带来重大损失,电话服务提供商采用了不同的规范来控制所购电信产品的可靠性和性能。在欧洲和东亚大部分地区,最常用的标准是ITU-T K.20和K.21。在北美地区,大多数运营公司的要求依据的都是包含GR 1089要求、TIA-968-A (旧称FCC第68部分)、UL 60950-1的NEBs。
本部分是对现有文件的说明,并未完整涵盖列出的建议、标准或监管要求。该信息仅作参考。确切规格请见 SIDACtor保护晶闸管规范要求,其中包括以下文档。
- 不同标准的浪涌波形
- GR 1089—核心
- ITU-T K.20和K.21
- TIA-968-A(旧称FCC第68部分)
- TIA-968-B(旧称FCC第6部分
- IEC 61000-4-2、4-4、4-5摘要
- 中国大陆标准——YD/T 950-1998
- 中国大陆标准——YD/T 993-1998
- 中国大陆标准——YD/T 1082-2000
- 中国国家认证认可监督管理委员会
- UL 497
- UL 497A
- UL 497B
- UL 497C
- UL 497D
- UL/IEC/EN 60950-1第2版
SIDACtor器件是晶闸管器件,用于保护敏感电路免受雷击感应浪涌、电感耦合尖峰和交流电源故障造成的电气干扰。我们利用晶闸管独特的结构和特性设计出了一款精度高、可重复开启的过电压保护器件,该器件具有低电压过冲和高浪涌电流能力。
关键参数
SIDACtor器件的关键参数为VDRM、IDRM、VS、IH和VT。
VDRM是器件的重复断态额定峰值电压(也称为断态电压),是可在SIDACtor器件处于断态状态时施加到交流和直流电压的连续峰值组合。
IDRM是应用VDRM产生的泄漏电流的最大值。
开关电压(VS)是后续元件在快速上升(100 V/μs)过压条件下可能承受的最大电压。
保持电流(IH)是使器件保持通态所需的最小电流。
通态电压(VT)是指完全导通期间器件上的最大电压。
工作原理
此器件的工作原理与开关十分相似。在关断状态下,该器件的泄漏电流(IDRM)小于5 μA,使其对所保护的电路不可见。当瞬态电压超过设备的VDRM时,设备开始进入保护模式,其特性类似于雪崩二极管。当电流(IS)足够大时,该器件将切换至导通状态,从而从所保护的电路中分流浪涌。由于器件两端的电压降(VT)较低,因此器件处于导通状态时能够吸收大量电流。一旦流经器件的电流中断或低于最小保持电流(IH),器件将复位并返回断态。如果超过IPP额定值,设备通常会永久短路。
Physics The device is a semiconductor device characterized as having four layers of alternating conductivity: PNPN (Figure 1.2 below).这四层分别是一个发射层、一个上基层、一个中间层和一个下基层。发射层有时也称为阴极区,下基层也称为阳极区。图 1.2 双向SIDACtor器件的几何结构
当器件两端的电压增加并超过器件的VDRM时,中心结两端的电场将达到足以引起雪崩倍增的值。在发生雪崩式倍增时,此器件的阻抗开始降低,电流增加,直到其电流增益超过1。超过1后,器件将从高阻抗(在VS下测得)切换到低阻抗(在VT下测得),直到流经器件的电流降至其保持电流(IH)以下。
The four most commonly used technologies for overvoltage protection are as follows:
- SIDACtor器件
- 气体放电管(GDT)
- 金属氧化压敏电阻(MOV)
- 瞬态抑制二极管
所有这四种装置都与所保护的电路进行并联,当偏压低于相应的断态重复峰值电压时都会表现出高断态阻抗。
SIDACtor器件
SIDACtor器件是一种PNPN器件,可以看作是没有门的晶闸管器件。当超过其峰值断态电压(VDRM)时,SIDACtor器件会将瞬态电压钳制在器件的开关电压(VS)额定范围内。一旦流经SIDACtor器件的电流超过其开关电流,器件将急剧短路,进入拟短路状态。当流经SIDACtor器件的电流小于器件的保持电流(IH)时,SIDACtor器件将复位并恢复到高断态阻抗的状态。
优点
SIDACtor器件的优点包括响应时间快(图1.1)、稳定的电气特性、长期可靠性和低电容。此外,由于SIDACtor器件是电撬器件,因此不会受到电压损坏。
局限性
由于SIDACtor器件是电撬器件,因此不能直接在交流线路上使用;它必须放在负载后面。否则将超过SIDACtor器件的最大通态电流额定值,从而导致器件进入永久短路状态。
应用
SIDACtor器件虽然也可用于其他应用,但主要用作电信和数据通信电路中的主要过压保护器。对于此领域之外的应用,请遵循SIDACtor器件选择标准中的设计标准。
气体放电管
气体放电管(GDT)采用玻璃或陶瓷封装,内部填充惰性气体,两端用电极封住。当瞬态电压超过器件的额定直流击穿电压时,电压差会造成气体放电管的电极着火,导致出现电弧,而电弧会造成管内气体离子化,并提供了一个让瞬态电压通过的低阻抗路径。当瞬态值降到直流过保持电压和电流以下时,气体放电管会返回到断态。
优点
气体放电管的浪涌电流高,额定电容低。在零伏偏压下,额定电流可高达20 kA,额定电容可低至1 pF。
应用
由于浪涌额定值高,气体放电管通常被用于基础保护。不过由于其对高频元件的干扰很低,它们也是高速数据链路的备选方案。
金属氧化物压敏电阻
金属氧化压敏电阻(MOV)是有两根引线的通孔元件,通常为圆盘形。MOV以烧结氧化物制成,大致相当于两个相接的PN结点,可通过在施加电压时降低电阻来对瞬态电流进行分流。
优点
由于MOV的抗浪涌能力由其外形尺寸所决定,故可提供高浪涌额定电流。此外,由于MOV属于箝制器件,因此可在次级交流电源线路中用作瞬态保护器。
应用
尽管MOV在很多电信设备(一次性设备除外)中都不能使用,但却可以用于需要箝制器件且不要求电压容差很小的交流电设备中。
瞬态抑制二极管
瞬态电压抑制器(TVS)二极管是由相接的PN结点构成的箝位电压抑制器。在导电过程中,当在其端子上施加电压时,瞬态抑制二极管会通过改变电阻来创建一个低阻抗的通道。一旦电压去除,二极管即会关闭并回到高断态阻抗的状态。
优点
由于瞬态抑制二极管是固态器件,因此只要在规定的范围内工作,就不会出现疲劳故障,其电气参数也不会发生变化。瞬态抑制二极管能够有效箝制迅速生成的瞬态电压,非常适合用于不需要对大量电能进行分流的低电压设备。
应用
由于额定功率低,瞬态抑制二极管不能用作正极线(Tip)和负极线(Ring)之间的初级接口保护器,但可用作嵌入电路中的次级保护器。
过冲级别与dv/dt
下图1.4显示了SIDACtor器件、气体放电管(GDT)、金属氧化物压敏电阻(MOV)和瞬态抑制二极管的峰值电压比较,两者的标称额定断态电压均为230 V。X轴表示施加到每个保护器上的dv/dt(电压随时间上升),Y轴表示每个保护器上的最大电压降。
图1.4 过冲级别与dv/dt
由于早期的电信设备是由机械继电器、线圈、真空管等元件构成的,它们在某种程度上不受雷击和电源故障的影响。不过随着步进开关和数字回路载体被更加现代化的设备(如多路复用器、路由器、网关和IP交换机)所取代,对于保护此类设备免受因雷击和电源故障造成的系统瞬态现象影响的需求也在日益增加。
雷击
在发生雷电风暴时,瞬态电压通过雷电流被导入电信系统,雷电流会进入空中电缆的导电屏蔽层,或通过大地电流进入埋在地下的电缆。
当发生这种情况时,流经电缆导电屏蔽层的电流会在正极线(Tip)和负极线(Ring)导体的末端产生同等的电压。这就是纵向电压浪涌,由此产生的峰值和波形由瞬态电流在电缆中传输的距离及电缆的制造材料所决定。
尽管由雷击导致的浪涌在本质上都属于纵向浪涌,但是,终端设备不平衡和初级保护器的不对称运行也可能产生金属瞬态浪涌。终端设备上经常发生从正极线(Tip)到负极线(Ring)的浪涌,这也是大多数监管机构要求为电信设备同时提供纵向和金属浪涌保护的主要原因。
电源故障
对于电信电缆来说,另一种常见的系统瞬态现象是由与交流电源系统接触而造成的。电极、沟槽、地线的一般使用会造成接触程度不一,可划分为直接电源故障、电力线感应和地电势上升。
当电源线与电信电缆直接接触时,会发生直接电源故障。直接接触通常是由树木倒落、冬季结冰、强雷暴天气和交通事故所导致。直接电源故障可导致线路中出现较大电流。
当电信电缆和供电电缆之间的距离很近时,通常会产生电力线感应。电缆间的电磁耦合可将系统瞬态电流导入电信电缆中,进而造成位于电缆末端的终端设备发生过热和起火。
地电势上升是大故障电流流入大地中的结果。由于土壤电阻率不同及存在多个接地点,因而可能会出现系统势差。
雷击
雷击是大自然中最常见的危险现象之一。全球大约随时发生着2,000场雷暴雨,其中每秒发生的雷击落地事件超过100次。据IEEE C.62统计,美国一年中平均每平方英里发生的雷击次数达到52次,造成100死、250伤及超过一亿美元的设备财产损失。
雷击现象
雷击是在常见的雷雨天气中由雨、冰、上升气流、下降气流的复杂交互作用产生的。云层中雨滴和冰雪的运动会造成大量电荷积聚在雷暴云的顶端和底端。通常情况下,正电荷集中在雷暴云的顶端,负电荷则集中在雷暴云的底端附近。当两端电荷的势差超过两端之间空气的绝缘电阻时,就会发生雷击。
雷击的形成
当较低云层中所包含的负电荷开始增加并吸引地面上的正电荷的时候,就会开始形成云层到地面的雷击。当负电荷的形成达到最大程度时,被称为“阶梯先导”的电子开始涌向地面。阶梯先导以50米的递增量移动,为雷击的发生准备好电通路(通道)。随着阶梯先导越来越接近地面,正电荷和负电荷之间的相互吸引作用会把正电子流从地面拉向阶梯先导。该正电荷流被称为电子流。当电子流和阶梯先导接触时即形成了云层和地面之间的完整电路。在这一瞬间,会有大量电子流以一半的光速涌向地面,完成闪电的形成。
闪电
当阶梯先导和电子流接触时会形成第一道闪电,将电流导向地面。随后,当大量负电荷继续沿阶梯先导上行时,会发生3至4次雷击。这些随后的闪电被称为回闪,将空气加热到50,000°F以上,并引起与雷击相关的闪烁闪光。大多数闪电的持续时间在500微秒到一秒之间。
在发生雷击时,相关电压范围为 20,000 V 至 1,000,000 V,电流平均约为 35 kA。然而,测得的与雷击相关的最大电流高达300 kA。
关于雷击的10大知识要点
- 平均每秒发生100次雷击落地事件。
- 雷击可对最远一公里以外的计算机和其他电子设备产生影响。
- 雷击会使电源、数据通信、信号和电话线路产生瞬态过电压(速度极快的电涌现象)。此类电涌会被传输至易损设备,对其造成影响。
- 易遭雷击的电子设备包括计算机和外围设备、楼宇管理系统、IP-PBX系统、CATV设备、火警和安防系统、PoE系统、照明阵列等。
- 瞬态过电压会造成设备和电路在瞬间损坏,进而导致损失严重的长时间运营中止和潜在损坏,并可在数周或数月后导致故障停机。
- 即便设备位于装有避雷设施的建筑物中也仍然面临很大风险,这是因为建筑中的避雷装置是用于防止对建筑物造成损坏以及保护人员生命安全的。
- 虽然大多数企业都面临风险,不过校园或拥有多个建筑物的场所更易遭受攻击。
- 雷击可能攻击同一地点(且确实发生过此类事件),并可多次攻击同一地点。若某些场所曾遭受过雷击,则说明这些场所易遭受雷击,且通常在数月之内会再次遭受雷击。
- 使用保护装置来保护电子系统免受瞬态过电压损坏所需的费用仅为损坏所造成损失的一小部分。
- Littelfuse设计并生产高质量的雷击保护设备。
- 不同标准的浪涌波形
- GR 1089—核心
- ITU-T K.20和K.21
- TIA-968-A(旧称FCC第68部分)
- TIA-968-A(旧称FCC第68部分)
- IEC 61000-4-2、4-4、4-5摘要
- 中国大陆标准——YD/T 950-1998
- 中国大陆标准——YD/T 993-1998
- 中国大陆标准——YD/T 1082-2000
- 中国国家认证认可监督管理委员会
- UL 497
- UL 497A
- UL 497B
- UL 497C
- UL 497D
- UL 60950-1 第2版